SimCor
SimCor is a nickname for Simultaneous correlation of vapor pressures ps and related thermal properties (enthalpies of vaporization/sublimation, and heat capacities of condensed and gaseous phases). SimCor is a powerful tool which can be used primarilly for two impotrant applications:
1) for testing consistency of involved properties (when temperature ranges of these properties overlap). See e.g. paper by Weber Criteria for establishing accurate vapour pressure curves [1]
2) for thermodynamically controlled extrapolation of vapor pressures (measured at higher temperatures) using thermal properties (measured/calculated at lower temperatures).
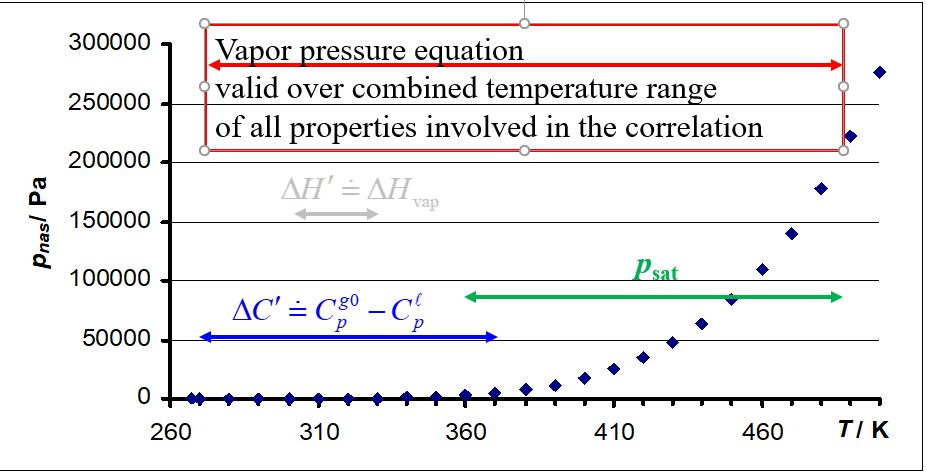
SimCor was suggested (in a simplified form) by King and Al-Najjar in 1974 [2,3]. The method was later sporadically used by several authors (e.g. by Ambrose and Davies [4], Mosselman et al. [5], Rogalski [6,7], King and Mahmud [8], Vetere [9] (who used simplified SimCor version with enthalpies of vaporization but without considering heat capacities), Bertucco et al. [10], Craven and de Reuck [11], Poling [12], Varushchenko et al. [13], Huber et al. [14], Hogge et al. [15, 16]) and systematically in our laboratory [19-55]. The SimCor is based on exact thermodynamic relationships and the procedure must therefore yield reliable results providing that the input data are of reasonable accuracy. A great advantage of this approach is that a single equation can furnish a description of the temperature dependences of several thermodynamic properties and the SimCor thus also provides a test on the consistency of different experimental data.
Thermodynamic background
A full description of the SimCor Method was presented previously by Růžička and Majer [21], however it is repeated here for reader's convenience. Moreover, detailed description of values used in the SimCor method for compounds of this study is provided.
Let us define auxiliary quantities ΔH' and ΔC':

The SimCor than starts from the Clapeyron equation in the form

which relates the vapor pressure p to Δcd,gHm and the pVT behavior of the coexisting phases. The differentiation yields an equation relating vapor pressures to heat capacities

It is apparent that quantities ΔH' and ΔC' can be calculated exclusively from vapor pressure equation (eqs 3 and 4) or from thermal properties (Δcd,gHm and Δcd,gCp,m ) and appropriate pVT corrections (eqs 1 and 2). This means that after selecting a suitable vapor pressure equation it is possible to correlate simultaneously experimental values of psat, Δcd,gHm and Δcd,gCp,m as a function of temperature, resulting in a set of vapor pressure equation parameters which are valid in a combined temperature range of all input experimental values.
As a result of SimCor, vapor pressure equation is valid over combined temperature renge of both heat capacities and vapor pressures entering the correlation as shown in the Figure above (vaporization enthalpy can be included, too).
Correction for nonideality of gaeous phase
Temperature/pressure limits for application of corrections for nonideality of gaseous phase
Thermal properties include corrections for nonideality of gaseous phase (eq 1 and 2 above, further on pVT corrections). At the normal boiling point temperature, this correction is about 5 percent in case of vaporization enthaply, but much larger ( ca 40 percent) for heat capacity differenc.
pVT correction can be negelected if the vapor pressure is sufficiently small. Heat capacity difference Δcd,gCp,m can be included in SimCor without pVT correction at temperatures corresponding to saturated pressures less than approximately 1o Pa; similarly enthalpies of vaporization Δcd,gHm can be included in SimCor without pVT correction at temperatures corresponding to saturated pressures less than approximately 1 kPa.
In case when pVT
correction is described by (estimated) second virial coefficient B, heat capacity difference
Δcd,gCp,m
can be included in SimCor at temperatures corresponding to saturated pressures
less than approximately 1 kPa; similarly enthalpies of vaporization Δcd,gHm
can beincluded in SimCor at temperatures corresponding to saturated pressures
less than approximately 10 kPa.
Above these limits, uncertainty in nonideality description could distort SimCor results.
Detailed description of corrections for nonideality of gaseous phase.
While calorimetry is a source of Δcd,gHm and Cp,m,cd , the heat capacity of real gas is obtained using

where Cp,m,g0 is isobaric heat capacity of ideal gas, evaluated usually from spectroscopic (or ab initio) vibrational frequencies by means of statistical thermodynamics, and Vm,g is molar volume of gas phase.
Generally,
the pVT term in equation (i.e. term
Δcd,gz
) represents correction around (3 to 7) percent to
Δcd,gHm
at the normal boiling
point temperature Tb,
while pVT terms in equation can amount up to 40 percent of
Δcd,gCp,m
value (see Fig. 1 bellow, originally from Růžička and Majer [21]).
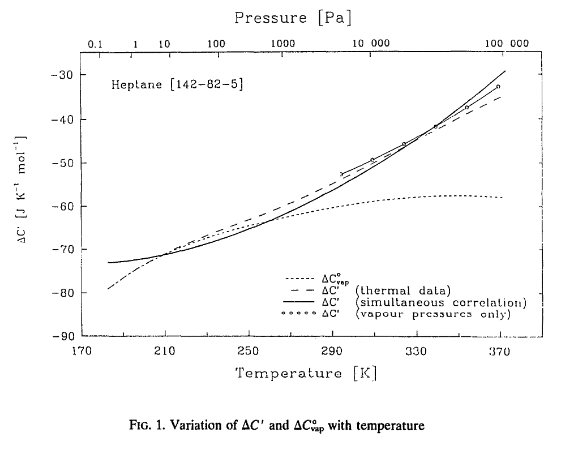
Since data for exact evaluation of pVT corrections are generally not available, all authors using the SimCor method had to select either of the two possible solutions:
- To neglect the pVT correction in equations 1 and 2 at all. This implies that enthalpies of vaporization/sublimation Δcd,gHm can be included in the SimCor at saturated pressures smaller than 1 kPa and heat capacity difference Δcd,gCp,m at saturated pressures less than 10 Pa. For example in the case of naphthalene [17] this corresponds to temperature of triple point temperature Ttr=353.37 K (p=993.5 Pa, pVT correction in eq 1 represents 0.19 %) and T=280 K (p=1.6 Pa, pVT correction in eq represents 0.17 %).
- To express the pVT correction by means of second virial coefficients (and molar volumes of liquid/solid phase). As experimental second virial coefficients are typically not available for temperatures well below the normal boiling temperature Tb, estimation methods must be used. This means that the uncertainty of pVT corrections is high and limits inclusion of thermal properties in the SimCor. Thus to avoid the distortion of SimCor by errors in pVT description, Δcd,gHm can be included in the SimCor at saturated pressures smaller than approximately 10 kPa and heat capacity difference Δcd,gCp,m at saturated pressures less than approximately 1 kPa
When the volume of gaseous phase is expressed as Vm,g = RT/p + B, eq 1 can be written as

and eq 4 can be converted (after neglecting the pressure dependence of Vm,cd ) to the form [2]

The molar volume of saturated condensed phase Vm,cd and its temperature derivative play negligible role at temperatures well below the normal boiling temperature, where equations 6 and 7 are applied.
Several
estimation methods for the second virial coefficient can be found in the
literature. We prefer the method suggested by Tsonopoulos [17], as
it provides also (empirical) corrections for polar compounds. Note that in the limits
suggested for applying pVT correction
(psat<10 kPa in eq
and psat<1 kPa in eq 7)
even large change of input parameters would not influence resulting vapor pressures
and enthalpies of sublimation.
Vapor pressure equations suitable for SimCor
Any vapor pressure equation can be used in the SimCor method providing it is flexible enough to describe input properties within their assumed uncertainties. Different equations were tested by Růžička and Majer [23]. The Cox equation [18] was used in most of our works as it is flexible and (in contrast to Wagner equation, which otherwise performs also quite well) does not require critical temperature and critical pressure.
The parameters of the vapor pressure equation are obtained by minimizing an objective function S, which is defined as

The quantities with the superscript "exp" relate to the experimental data (ΔH'exp are calculated by means of eq 6 and ΔC'exp by means of eq 7). The quantities with the superscript "calc" are expressed from the Cox equation and eqs 3 and 4. The individual data points are weighted using the expected uncertainties of the experimental data. As a starting point, uncertainties claimed in the original data source are used. Very often, the original uncertainties are too optimistic and they are raised during SimCor or the whole dataset is even excluded from the correlation, when inconsistency with other types of data is observed. The quantity σi^2 ln psat is obtained from errors in temperature (σ T) and pressure (σ p)

.
σi^2 ΔH' is evaluated from the
uncertainty in the enthalpy of vaporization/sublimation as
σi^2 ΔH' = (σΔcd,gHm)^2.
It is usually set to 5 percent for vaporization enthalpy obtained by drop
calorimetry, which is included in SimCor.
Finally, σi^2 ΔC' is estimated from the expected uncertainties in heat capacities of respective phases

σCp,m,cd for values included in SimCor calculated from a polynomic equation fit are set to 1 percent for data from Tian-Calvet calorimeters and 0.3 percent for data from adiabatic calorimeters. σCp,m,g is set to 1 percent for Cp,m,g based on ab-initio vibrational frequencies. Weighing factors KH^2 and KC^2 are set to unity unless inconsistency between the different types of data is observed. Whenever possible, the inconsistency is resolved by new measurements instead of by changing KH^2 and KC^2.
References
[1] L. A.
Weber, Criteria for establishing accurate vapour pressure curves. International Journal of Refrigeration 1994, 17, 117-122.
[2] M.B. King, H.
Al-Najjar, Method for correlating and extending vapor pressure data to lower
temperatures using thermal data. Vapor pressure equations for some n-alkanes at temperatures below the
normal boiling point, Chem. Eng. Sci., 29 (1974) 1003-1011.
[3] M.B. King, Correlation, Prediction and Extrapolation of Vapor-Pressure and Related Thermal Data, Trans. Inst. Chem. Eng., 54 (1976) 54-60.
[4] D. Ambrose, R.H. Davies, The correlation and estimation of vapor pressures. III. Reference values for low-pressure estimations, J. Chem. Thermodyn., 12 (1980) 871-879.
[5] C. Mosselman, W.H. Van Vugt, H. Vos, Exactly integrated Clapeyron equation. Its use to calculate quantities of phase change and to design vapor pressure-temperature relations, J. Chem. Eng. Data, 27 (1982) 246-251.
[6] M. Rogalski, Correlation of vapor pressures of pure substances in homologous series, Thermochim. Acta, 90 (1985) 125-133.
[7] B. Wiśniewska, M. Łencka, M. Rogalski, Vapour pressures of 2,4-, 2,6-, and 3,5-dimethylpyridine at temperatures from 267 to 360 K, J. Chem. Thermodyn., 18 (1986) 703-708.
[8] M.B. King, R.S. Mahmud, A reduced vapour pressure equation for use at low reduced temperatures, Fluid Phase Equilib., 27 (1986) 309-330.
[9] A. Vetere, Methods for predicting and correlating the vapour pressures of pure compounds, Fluid Phase Equilib., 43 (1988) 191-203.
[10] A. Bertucco, R. Piccinno, G. Soave, A method for estimating vapour pressures of pure high boiling substances, Chem. Eng. Commun., 106 (1991) 177-184.
[11] R.J.B. Craven, K.M. de Reuck, Development of a vapour pressure equation for methanol, Fluid Phase Equilib., 89 (1993) 19-29.
[12] B.E. Poling, Vapor pressure prediction and correlation from the triple point to the critical point, Fluid Phase Equilib., 116 (1996) 102-109.
[13] R.M. Varushchenko, L.L. Pashchenko, A.I. Druzhinina, A.V. Abramenkov, A.A. Pimersin, Thermodynamics of vaporization of some alkyladamantanes, J. Chem. Thermodyn., 33 (2001) 733-744.
[14] M.L. Huber, A. Laesecke, D.G. Friend, Correlation for the Vapor Pressure of Mercury, Ind. Eng. Chem. Res., 45 (2006) 7351-7361.
[15] J.W. Hogge, N.F. Giles, T.A. Knotts, R.L. Rowley, W.V. Wilding, The Riedel vapor pressure correlation and multi-property optimization, Fluid Phase Equilib., 429 (2016) 149-165.
[16] J.W. Hogge, R. Messerly, N. Giles, T. Knotts, R. Rowley, W.V. Wilding, Improving thermodynamic consistency among vapor pressure, heat of vaporization, and liquid and ideal gas isobaric heat capacities through multi-property optimization, Fluid Phase Equilib., 418 (2016) 37-43.
[17] C. Tsonopoulos, Empirical correlation of second virial coefficients, AIChE J., 20 (1974) 263-272.
[18] E.R. Cox, Hydrocarbon vapor pressures, J. Ind. Eng. Chem., 28 (1936) 613-616.
[19] K. Růžička, M. Fulem, V. Růžička, Recommended Vapor Pressure of Solid Naphthalene, J. Chem. Eng. Data, 50 (2005) 1956-1970.
[20] K. Růžička, V. Majer, A simultaneous correlation of vapor pressures and thermal data: application to 1-alkanols, Fluid Phase Equilib., 28 (1986) 253-264.
[21] K. Růžička, V. Majer, Simultaneous treatment of vapor pressures and related thermal data between the triple and normal boiling temperatures for n-alkanes C5-C20, J. Phys. Chem. Ref. Data, 23 (1994) 1-39.
[22] V. Růžička, M. Zábranský, K. Růžička, V. Majer, Vapor pressures for a group of high-boiling alkylbenzenes under environmental conditions, Thermochim. Acta, 245 (1994) 121-144.
[23] K. Růžička, V. Majer, Simple and controlled extrapolation of vapor pressures toward the triple point, AIChE J., 42 (1996) 1723-1740.
[24] K. Růžička, I. Mokbel, V. Majer, V. Růžička, J. Jose, M. Zábranský, Description of vapor-liquid and vapor-solid equilibria for a group of polycondensed compounds of petroleum interest, Fluid Phase Equilib., 148 (1998) 107-137.[25] V. Roháč, J.E. Musgrove, K. Růžička, V. Růžička, M. Zábranský, K. Aim, Thermodynamic properties of dimethyl phthalate along the (vapour + liquid) saturation curve, J. Chem. Thermodyn., 31 (1999) 971-986.
[26] V. Roháč, V. Růžička, K. Růžička, M. Poledníček, K. Aim, J. Jose, M. Zábranský, Recommended vapor and sublimation pressures and related thermal data for chlorobenzenes, Fluid Phase Equilib., 157 (1999) 121-142.
[27] I. Mokbel, K. Růžička, V. Majer, V. Růžička, M. Ribeiro, J. Jose, M. Zábranský, Phase equilibria for three compounds of petroleum interest: 1-phenyldodecane, (5alpha)-cholestane, adamantane, Fluid Phase Equilib., 169 (2000) 191-207.
[28] I. Cibulka, T. Takagi, K. Růžička, P-r-T data of liquids: summarization and evaluation. 7. Selected halogenated hydrocarbons, J. Chem. Eng. Data, 46 (2001) 2-28.
[29] V. Roháč, K. Růžička, V. Růžička, D.H. Zaitsau, G.J. Kabo, V. Diky, K. Aim, Vapour pressure of diethyl phthalate, J. Chem. Thermodyn., 36 (2004) 929-937.
[30] M. Fulem, K. Růžička, M. Růžička, Recommended vapor pressures for thiophene, sulfolane, and dimethyl sulfoxide, Fluid Phase Equilib., 303 (2011) 205-216.
[31] M. Fulem, K. Růžička, C. Červinka, M.A.A. Rocha, L.M.N.B.F. Santos, R.F. Berg, Recommended vapor pressure and thermophysical data for ferrocene, J. Chem. Thermodyn., 57 (2013) 530-540.
[32] V. Štejfa, M. Fulem, K. Růžička, C. Červinka, M.A.A. Rocha, L.M.N.B.F. Santos, B. Schröder, Thermodynamic study of selected monoterpenes, J. Chem. Thermodyn., 60 (2013) 117-125.
[33] V. Štejfa, M. Fulem, K. Růžička, C. Červinka, Thermodynamic study of selected monoterpenes II, J. Chem. Thermodyn., 79 (2014) 272-279.
[34] M. Fulem, K. Růžička, C. Červinka, A. Bazyleva, G. Della Gatta, Thermodynamic study of alkane-α,ω-diamines - Evidence of odd-even pattern of sublimation properties, Fluid Phase Equilib., 371 (2014) 93-105.
[35] K. Růžička, M. Fulem, C. Červinka, Recommended sublimation pressure and enthalpy of benzene, J. Chem. Thermodyn., 68 (2014) 40-47.
[36] V. Štejfa, M. Fulem, K. Růžička, C. Červinka, Thermodynamic study of selected monoterpenes III, J. Chem. Thermodyn., 79 (2014) 280-289.
[37] K. Růžička, M. Fulem, T. Mahnel, C. Červinka, Recommended vapor pressures for aniline, nitromethane, 2-aminoethanol, and 1-methyl-2-pyrrolidone, Fluid Phase Equilib., 406 (2015) 34-46.
[38] V. Štejfa, F. Dergal, I. Mokbel, M. Fulem, J. Jose, K. Růžička, Vapor pressures and thermophysical properties of selected monoterpenoids, Fluid Phase Equilib., 406 (2015) 124-133.
[39] V. Štejfa, M. Fulem, K. Růžička, P. Matějka, Vapor pressures and thermophysical properties of selected hexenols and recommended vapor pressure for hexan-1-ol, Fluid Phase Equilib., 402 (2015) 18-29.
[40] V. Štejfa, M. Fulem, K. Růžička, P. Morávek, New Static Apparatus for Vapor Pressure Measurements: Reconciled Thermophysical Data for Benzophenone, J. Chem. Eng. Data, 61 (2016) 3627−3639.
[41] T. Mahnel, V. Štejfa, M. Fulem, K. Růžička, Recommended vapor pressures for acenaphthylene, fluoranthene, and fluorene, Fluid Phase Equilib., 434 (2017) 74-86.
[42] V. Pokorný, V. Štejfa, M. Fulem, C. Červinka, K. Růžička, Vapor pressures and thermophysical properties of dimethyl carbonate, diethyl carbonate, and dipropyl carbonate, J. Chem. Eng. Data, 62 (2017) 3206-3215.
[43] Soares B.P.; Štejfa V.; Ferreira O.; Pinho S.P.; Růžička K.; Fulem M.,
Vapor pressures and thermophysical properties of selected
ethanolamines. Fluid Phase Equilibria, 2018, 473, 245-254.
[44] Mahnel T.; Štejfa V.; Maryška M.; Fulem M.; Růžička K., Reconciled thermophysical data for anthracene. Journal of Chemical Thermodynamics, 2019, 129, 61-72. https://doi.org/10.1016/j.jct.2018.08.034
https://doi.org/10.1021/acs.jced.8b01159
[46] Štejfa V.; Bazyleva A.; Fulem M.; Rohlíček J.; Skořepová E.; Růžička K.; Blokhin A. V., Polymorphism and thermophysical properties of L- and DL- menthol. Journal of Chemical Thermodynamics, 2019, 131, 524-543. https://doi.org/10.1016/j.jct.2018.11.004
[47]
Vilas-Boas
S. M.; Pokorný V.; Štejfa V.;
Ferreira O.; Pinho S. P.; Růžička K.;
Fulem M., Vapor pressure and thermophysical properties of eugenol and (+)-carvone. Fluid
Phase Equilibria, 2019, 499, 112248.
https://doi.org/10.1016/j.fluid.2019.112248
[48] Štejfa V.; Fulem M.; Růžička K.; Thermodynamic study of selected monoterpenes IV,
The Journal of Chemical Thermodynamics, 144, 2020, 106013 https://doi.org/10.1016/j.jct.2019.106013
[49]. Štejfa V., Mahnel T., Skořepová E., Rohlíček J., Eigner V., Schröder B., Růžička K., Fulem M.
A combined thermodynamic and crystallographic study of 1,3-diisopropylnaphthalene.
The Journal of Chemical Thermodynamics, 150, 2020, 106193, https://doi.org/10.1016/j.jct.2020.106193
[50] Štejfa, V.; Chun, S.; Pokorný, V.; Fulem, M.; Růžička, K., Thermodynamic study of acetamides. J. Mol. Liq. 2020, 114019. https://doi.org/10.1016/j.molliq.2020.114019
[51] Štejfa
V., Kadlecová K., Růžička K., Fulem M. Vapor pressure and thermophysical properties of explosive taggants
Chemical Thermodynamics and Thermal Analysis, 3-4,(2021) 100020. https://doi.org/10.1016/j.ctta.2021.100020
[52] Pokorný
V.; Štejfa V.;
Pavlíček J.; Klajmon M.; Fulem M,; Růžička K., Vapor Pressures and Thermophysical Properties of Dimethoxymethane,
1,2-Dimethoxyethane, 2-Methoxyethanol, and 2-Ethoxyethanol: Data Reconciliation
and Perturbed-Chain Statistical Associating Fluid Theory Modeling. Journal of Chemical & Engineering Data, 2021, 66(6) 2640-2654. DOI: https://pubs.acs.org/doi/10.1021/acs.jced.1c00229
[53] Pokorný
V.; Štejfa V.;
Klajmon M.; Fulem M,; Růžička K., Vapor Pressures and Thermophysical Properties of of 1-Heptanol, 1-Octanol,
1-Nonanol, and 1-Decanol: Data Reconciliation and PC-SAFT Modeling, Journal of Chemical & Engineering Data, 2021, 66(1) 805-821. DOI: https://doi.org/10.1021/acs.jced.0c00878
[54] Zalčík, A.; Štejfa, V.; Fulem, M.; Růžička, K., Vapor pressures and thermophysical properties of selected monoterpenoids II. Fluid Phase Equilibria 2023, 567, 113704. https://doi.org/10.1016/j.fluid.2022.113704
[55] Štejfa V, Fulem M, Růžička
K. Thermodynamic study of selected aromatic monoterpenoids.
Journal of
Molecular Liquids. 2023 380 121724. https://doi.org/10.1016/j.molliq.2023.121724